Aerospace Applications
Composite structures provide the aerospace industry with increased aircraft range, strength, and safety while reducing development and manufacturing lead times and costs. Computational models and simulations of composite structures are inherently difficult because of the highly-engineered and non-homogeneous structures within the composite parts.
MARS is a very effective tool for modeling composite structures. Composites are heterogeneous material where damage predominately occurring in the mesoscale range becomes the dominant influence on the ultimate failure modes.
The DM4C module explicitly simulates composite fiber deposition and matrix polymerization, enabling an ultra-high-fidelity model of the bond formation at the mesoscale level. As a result, the ultra-high-fidelity MARS models which mirror the physical composition of the composite material can then be used to computationally simulate and predict the composite’s failure modes. Here are a few examples:
Longitudinal Compression Test with Kink Band Failure
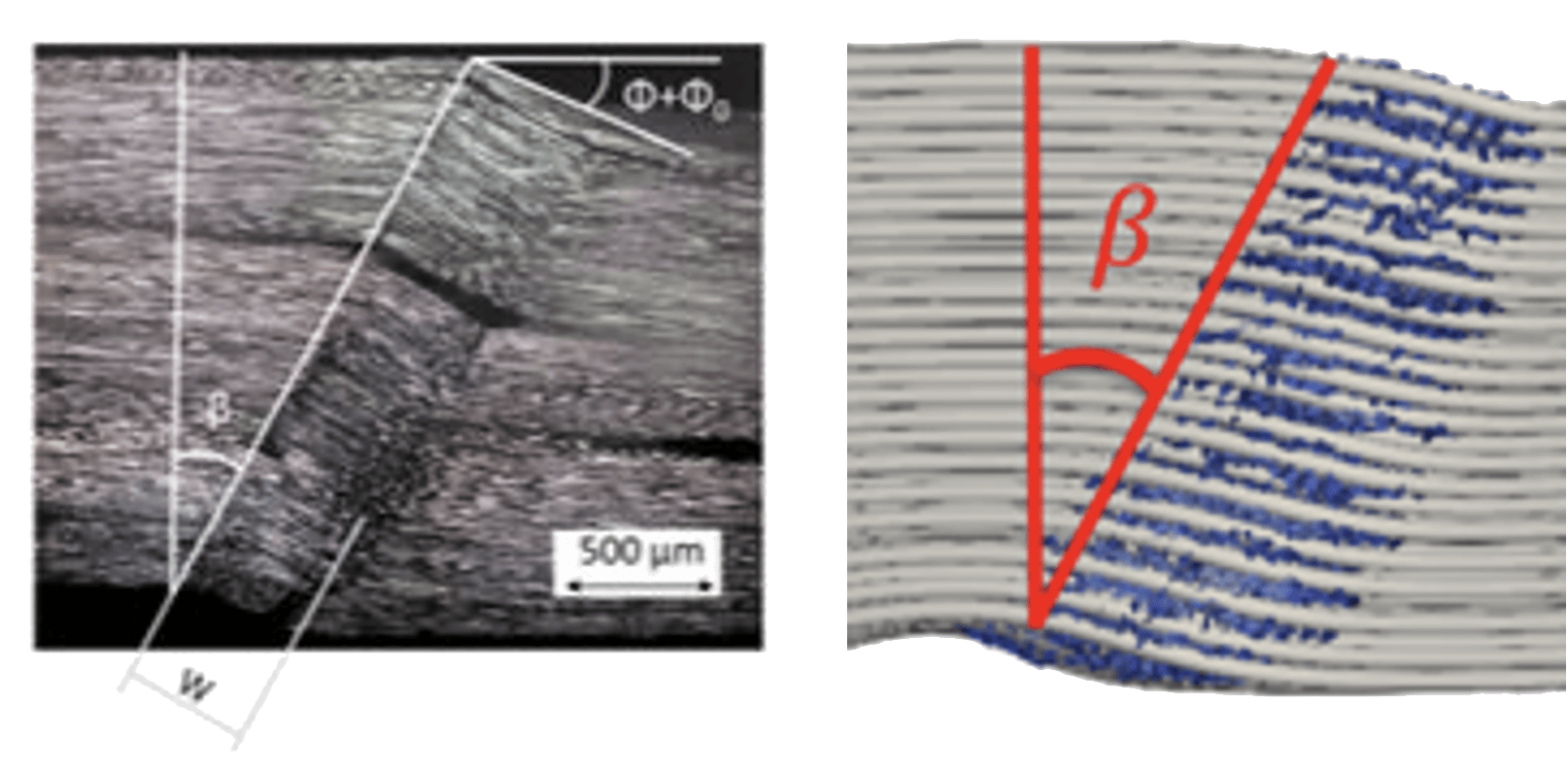
The MARS model and computational simulation accurately predicts the actual kink band failure modes of the buckling fibers. Provided is a video of the MARS simulated longitudinal compression test. The damage becomes visible eight seconds into the video.
Transverse Compression Tests
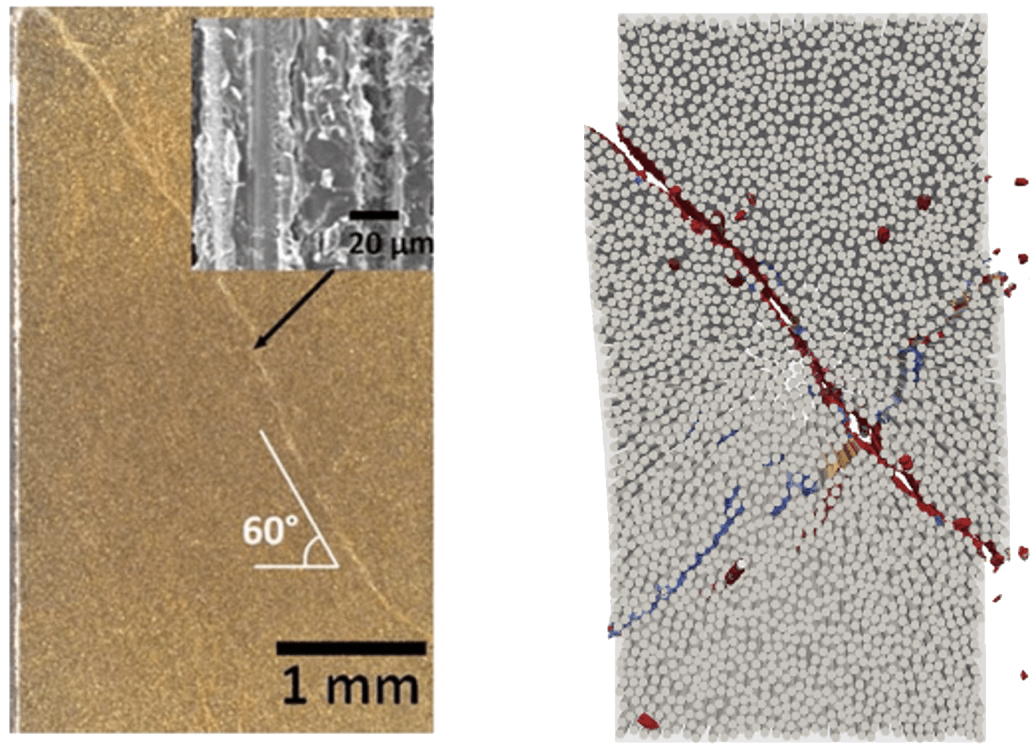
The MARS model and computational simulation accurately predicts the ultimate crack failure mode of the composite material. Provided is a video of the MARS simulated transverse compression test. The damage become visible ten seconds into the video.
Digital Engineering
MARS physics-based modeling can reduce the number of physical tests required to explore a variety of material designs. For example, each manufacturing approach exhibits its own set of signature defects. Moreover, even for the same manufacturing approach, changes in processing conditions such as but not limited to carbon material, bond material, print speed, nozzle / tool temperatures, tow size, tow path, and radius of curvature can lead to substantially different material performance. Relying solely on physical tests to engineer a composite aerospace part would be costly and time consuming. Instead, digital engineering can more quickly consider and fine-tune design options.
Digital engineering was used to develop a composite joint design for a US Air Force application.